Rose petals for solar power
And yet again, nature shows the way to make the best use of sunlight, says S.Ananthanarayanan.
The solar cell takes in light energy to and turns it into electricity. Vegetation makes use of light energy too, but to drive chemical changes to build carbohydrates. While the solar cell is a recent entrant, plants have been in the business since ages
and have evolved to capture and use as much of the light that falls on them as possible.
Ruben Hünig, Adrian Mertens, Moritz Stephan, Alexander Schulz, Benjamin Richter, Michael Hetterich, Michael Powalla, Uli Lemmer, Alexander Colsmann, and Guillaume Gomard, from Karlsruhe Institute of Technology, in Karlsruhe, Germany and the Centre for
Solar Energy and Hydrogen Research, Stuttgart, report in the journal, Advanced Optical Materials, that they have been able to isolate and reproduce the surface structure of the petals of the garden rose to create a surface that helps solar cells reduce how
much light they lose to reflection, and hence a 13% increase in their efficiency.
The authors explain that an early instance of an anti-reflecting surface, which was studied, is that of the eye of the moth, which needs both to see in the dark as well as to stay out of sight of predators. The moth’s eye surface, or the cornea, is provided
with an array of protrusions, of the dimensions of the wavelength of light, which make for a great part of light falling on the surface to pass through, in place of being reflected and lost. While this feature of the moth is effective to admit more light
and also show no reflection, plant surfaces can go a step further, by also rearranging the light waves that they pass without so that the waves can be better used by the underlying light mechanism, the authors say.
Light, as we know, consists of a combination of electric and magnetic waves, each being the cause and also the effect of the other, as they pass through, vacuum, in space, or the air, or glass, or water, etc. We can imagine that passage of the waves,
particularly their speed, depends on how electric or magnetic fields behave in different media. And in the case of all media, the effect is that the speed of light is less than what it is in vacuum, and for most materials, less than what it is in air. This
is why a light beam bends when it enters glass or water, and it is thanks to this that we have cameras, telescopes and the magnifying glass.
Apart from affecting the speed and the direction of light, these properties of materials also affect how much of incident light would pass through or reflect off a surface. For all materials, hence, a part of the light that falls on a surface is not transmitted,
but is reflected. This is so for almost all the angles at which light strikes the surface, with more reflection when the angle is shallow. Some of the light is thus always lost to reflection by the normal cornea of the eye, the leaf surface, which needs light
to create sugars, and, what is vital now for industry, the surface of the photo cell. And hence the great interest and value in developing ‘anti-reflecting’ or AR surfaces, to capture all the light falls on them.
In taking up the study of AR capabilities in plants, the researchers first carried out a survey of different plant surfaces to see which one was the best. The result of the survey is shown in the graph in the top left in picture 1, which shows how the
proportion of reflection drops as we move from a plane glass sheet to the surface of different kinds of leaf or petal, and reflection is the least, about 8%, in the case of the rose petal.
As shown in the inset on the top right of the same picture, the surface of the rose petal has protrusions packed close together, some 19 microns high and 32 microns wide, or nearly a half wider than they are high.
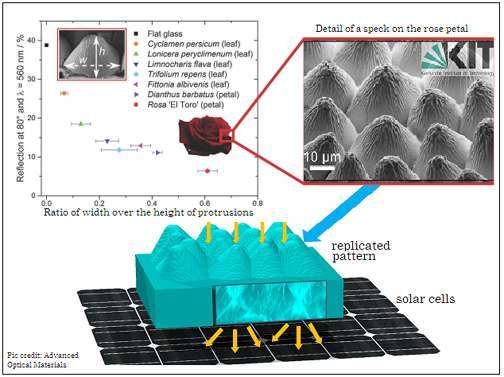
The experimenters copied the micro-pattern of the petals’ surface onto a silicone polymer mould. The mould was then used to create the same pattern on the surface of a glass substrate, as in the lower half, picture 1, The reflection properties of this
surface were then compared with those of a similar glass sheet with a simple cover with no micro-pattern. As shown in the graph in picture 2, while the level of reflection is low and about equal when light fell directly on the surfaces, this changes as the
slant increases and, at glancing angles, the level of reflection is many times lower for the rose structure treated surface. The trials also showed that this was so over all colours of the spectrum, which makes the rose petal structure a very attractive template
for light collection.
The study of the micro-pattern on the surface of the moth cornea, published in the year 2006, includes a discussion of the reason why the micro-pattern reduces the extent of reflection. As stated earlier, the reflection of light waves from transparent
surfaces happens because of the difference of the speed of light in vacuum, or air, and the material whose surface it is. It is the steep change in the material properties, and hence the speed of light, when light moves from vacuum or air to other materials,
that brings about reflection rather than passage through the transparent material. This is where the microstructure comes in. As the structures are of the same dimensions as the wavelength of light, they are able to affect the speed of light, and as the waves
move from the peaks of the micro-protuberances to their base, they experience a gradual change of speed, in place of the sudden change when they strike a plane surface. For the same reason, the pattern on the rose petal, now transferred to the experimental
glass sheet, is able to ease the transition from air to glass and reduce the proportion of light that is reflected.
The study also showed that the rose petal structure, which behaved like an array of micro-lenses, had the effect of making light diverge within the underlying medium, a light focusing effect, which increased the extent of its path and the efficiency of
conversion in a solar cell. In trials that were carried out with organic solar cells, the experimenters report an efficiency rise of 13% when the rose petal structure was used. This was with light falling normally on the solar cell. At glancing angles, the
rise in efficiency was as high as 44%.
“While standard micro lens arrays show both AR and light trapping properties, the rose structure enhances these effects through auxiliary nanofoldings…” the authors of the paper say. Further, with natural structures, higher ‘width to height’ ratios are
possible, apart from the ease of fabrication by simple replication, they say. There is need to gain “further knowledge about the contribution of the nanofolding, in particular its impact on the broadening of the propagation angle distribution,” the authors
say.
[the writer can be contacted at response@simplescience.in]
More help for the solar cell
The moth, which forages at night, is an evolutionary predecessor of butterflies, which are colourful and active in daylight. Butterflies appear to have lost the light capturing feature of the moth cornea. But there is a species of butterfly that has a
feature that is useful in the application of solar cells.
As the butterflies need to conserve weight, they have lightweight wings and limited muscular resources. The muscles used to take to flight hence need to warm in the sun before they can be used. The White Pieris butterfly, however, is found to get started,
even on cloudy days, before other kinds of butterfly.
The reason is the angle at which it holds its wings, but mainly a nano-pattern that covers the surface of the wings. Striations with spacing of the order of the wavelength of light, ruled on a reflecting surface, can focus light. Each wavelength, however
focuses at a different angle, and this may not be useful to collect sunlight, which has many wavelengths. And a random pattern would not be of any use at all. But a ‘quasi-random’ pattern, which can be painstakingly generated on a computer, it is found, can
focus a range of wavelengths. Now, the White Pieris butterfly has evolved to have just this kind of pattern on its wings. The wings thus focus all the use useful wavelengths of sun’s warmth on to the Pieris’ muscles and it takes to flight before others can.
The pattern on the wings have been peeled off and used with solar cells, resulting in huge increase in output, and also with addition of very little weight.
Another development is that the patterns on Blu Ray discs, which are a complicated coding of text matter or images, also have a quasi random character. These have also been found good for focusing a range of wavelengths on to solar cells, with the advantage
that they can be mass-produced.
While these are methods of getting more light to fall on the solar cell, the Karlsruhe/Stuttgart-rose petal advance is to help the solar cell make the best of the light that does.