Parallel computing to tame biofuel
The 21st century may belong to both bioscience and the power of computing, says S.Ananthanarayanan.
In a warming world, biological processes would be the viable way to provide food, as well as materials for non-food applications. And it is with the help of increasing computing ability that the world may be able to manage the growing complexity of demand
and supply while keeping the drain on the earth’s resources under control.
A team at the Oak Ridge National Laboratory, Tennessee has pressed into service the massive computing potential of the supercomputer, Titan, the first, in 2013, with the capacity of 10 petaflops, or ten million times a billion decimal calculations every
second, to understand the mechanics of a roadblock in one of the most urgent and promising solutions that bioscience may be able to provide to replace fossil fuels.
The solution in question is the production of ethanol, or alcohol, from biomass, or plant tissue, to power all kinds of machinery and transport, which now depend on petroleum, and possibly for generating electricity, by replacing coal as the fuel for power
plants. And the roadblock is that the capacity to get ethanol out of biomass is severely limited by lignin, a natural constituent of plant material itself.
The most common method of getting ethanol from plant material is by fermentation of the sugars in fruit or sugars derived from starch in grain. Ethanol is produced for industry from cane sugar or from corn that is grown for producing ethanol. While cane
sugar can be fermented directly, the starch in corn is first converted to sugars by the action of enzymes and the sugars are then fermented, and the ferment is distilled to get the required grade of alcohol. The commercially attractive proposition, however,
is not from cane or from corn, it is from the inedible, cellulose material in plant tissue. The advantage with cellulosic ethanol is that cellulose is abundant without having to be specially cultivated, so that its use does not affect the land available for
growing food grain. A major negative is the extra cost in the pretreatment and special enzymes that are needed to break cellulose into fermentable sugars, but the economics are still in its favour. The table shows the advantage of ethanol from corn or cellulose,
over gasoline, along with the case of what happens if land is used exclusively to grow cellulose.
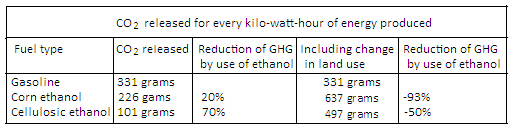
We can see that there is positive saving of GHG emissions with the use of ethanol. This is despite the negatives in the last column, because cellulose actually does not need to be cultivated specially, as it is abundant in unused plant waste, paper and packaging
waste and wood shavings and in good quantity from woody forest waste. There is more cellulose, in fact, than any other organic molecule on earth!
The problem
The function of cellulose, which forms the bulk of the plant cell wall, is to provide both protection and a rigid framework for the plant to stand and receive air and sunshine, and for channels that allow movement of nutrients.
The cellulose fibre alone, however, does not have the necessary rigidity, and it derives strength from lignin, a complex, chain molecule that forms the scaffolding on which cellulose makes up the cell wall. Hardier plant tissue, like the bark of trees, is
particularly rich in lignin, which is resilient and helps plant tissue combat corrosion and weathering. The trouble is that when plant tissue is made use of for the non-plant purpose of being broken down to sugars for fermentation, its lignin contents continue
to exercise their protective role.
The first step in processing biomass is heating with dilute acid, to remove most of the non-cellulose components of biomass, which is generally successful, except for the lignin content, which persists. The objective is to increase the access to cellulose
of the enzymes that convert cellulose to sugars. But even after this treatment, the conversion remains incomplete and the suspicion has been that it is the presence of lignin that is responsible. Some ways of how this comes about could be cellulose associating
with lignin in a way that the enzyme is prevented from acting on cellulose or lignin binding with the enzyme to disable its action, says a paper by Jeremy C Smith, professor at the University of Tennessee (UT) and director of the oak Ridge National Laboratory
Center for Molecular Biophysics, with Josh V Vernaas, Loukas Petridas, Xianghong Qi, Ronald Schulz and Benjamin Lindner, in the journal, Biotechnology for biofuels. Evidence for the hypothesis or about the mechanism of its action, however, has not been available,
the paper says.
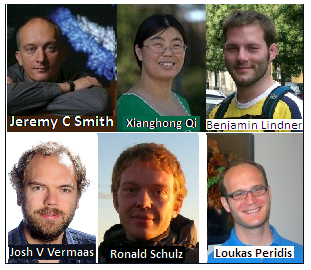
Computer simulation
As both cellulose and lignin are polymers, or molecules where a pattern repeats hundreds of times, and the chain winds and unwinds millions of times a second in the ceaseless motion at the atomic scale, experimental investigation into how lignin actually
inhibits the action of enzymes is not practical. The team at ORNL hence created a computer simulation to represent the interactions, to try out and analyse the billions of orientations of atoms.
For a fair representation of the problem, the simulation needed to consider as many as 23.7 million atoms and the computer had to take into account the orientations and interactions of the mechanical and electrical forces that act in this huge complex
of cellulose, lignin and the added enzymes. The modeling was limited to basic forms of the cellulose chains and the commonly encountered lignin molecules, and other parameters were based on available experiential data. Established algorithms, or computer procedures
to mimic the interactions, and available software packages were then used to analyse the huge data arising from simulation of even a few seconds of the atomic scale process.
As each instant of the process involved computation of millions of atoms, the processing was carried out with the help of Titan, the mega, parallel processing computer system at the Oak Ridge laboratory. Dealing with huge computations, as one which involves
millions of atoms, can take years if the operations are done one after another. Titan hence splits the work into independent sections and carries them out simultaneously with the help of effectively over 18,000 separate computers working together. As can be
imagined, this also needs software to separate the parallel procedures in the virtual model of the atomic system and then to put things together, for each instant of the interaction being modeled. Each of the runs of the simulation hence covered very short
stretches of actual process, the longest being 45 nanoseconds and the total over the course of a year has come to 1.3 microseconds. This much time, however, represents a viable collection of information of a process that occurs very rapidly and it has been
possible to piece together a useful picture of how lignin affects the interaction of cellulose and enzymes.
The results of the analyses were to confirm the suspicions, but with detail at the atomic level, that lignin forms bonds with cellulose at the very places where the enzymes would need to dock. This by itself impedes or limits the action of enzymes. But
lignin goes further - it forms bonds with the enzyme at the places in the structure of the enzyme which are needed for binding with cellulose. In this way, the action of the enzyme is hit twice – the targets are blocked and where they may be free, the enzyme
itself is disabled.
Another detail found through the simulation is that lignin forms bonds more readily with a crystalline form of cellulose than with a non-crystalline form. The knowledge of the molecular mechanism of lignin action and the preference for the crystalline
form could lead to processes, on the one hand, for engineering the form of lignin so that it bonds less with cellulose, and on the other, to favour production of non-crystalline cellulose during pretreatment. ”Eventually, we would like to construct a simple
model of a plant cell wall that we could process in silico, or via computer simulation, and see how it changes during pretreatment," says Loukas Petridis, staff scIentist at the Center for Molecular Biophysics," we're trying to reach the complexity that is
found in nature and industrial conditions.”
Do respond to : response@simplescience.in